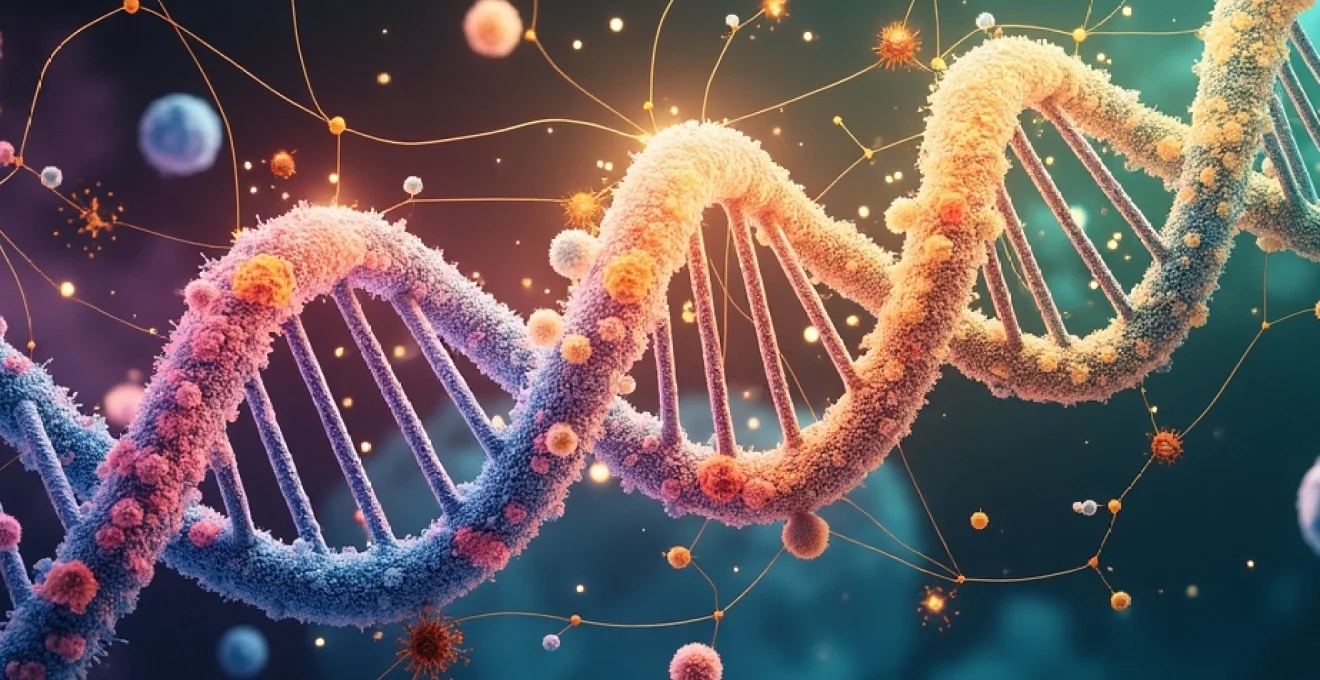
Rare diseases affect millions of people worldwide, yet many remain without effective treatments or cures. The key to unlocking solutions for these conditions lies in cutting-edge research and innovative approaches. From genomic sequencing to high-throughput drug screening, collaborative initiatives to precision medicine, the field of rare disease research is advancing rapidly. This progress offers hope to patients and families affected by these often debilitating conditions, while also pushing the boundaries of medical science and drug development.
Genomic sequencing techniques for rare disease identification
Genomic sequencing has revolutionized the field of rare disease research, offering unprecedented insights into the genetic basis of these conditions. By analyzing an individual's DNA, researchers can identify mutations that may be responsible for rare disorders, leading to more accurate diagnoses and potential treatment targets.
Whole exome sequencing (WES) in rare disease diagnosis
Whole Exome Sequencing (WES) has emerged as a powerful tool in the diagnosis of rare genetic disorders. This technique focuses on sequencing only the protein-coding regions of the genome, known as exons, which contain about 85% of known disease-causing mutations. WES is particularly useful in rare disease research because it can identify novel mutations in genes that have not previously been associated with a particular condition.
By analyzing the exome, researchers can often pinpoint the genetic cause of a rare disease in cases where traditional diagnostic methods have failed. This not only provides answers for patients and families but also opens up new avenues for targeted therapies. The cost-effectiveness and relatively quick turnaround time of WES make it an increasingly popular choice in both research and clinical settings.
Single-cell RNA sequencing for rare cell type analysis
Single-cell RNA sequencing (scRNA-seq) is a cutting-edge technique that allows researchers to study gene expression at the individual cell level. This approach is particularly valuable in rare disease research, where affected cell types may be present in very small numbers or mixed with healthy cells.
By examining the transcriptome of individual cells, scientists can identify rare cell populations and understand how they differ from normal cells. This level of detail can reveal new insights into disease mechanisms and potential therapeutic targets. For example, scRNA-seq has been used to study rare immune cell subsets in autoimmune disorders and to characterize tumor heterogeneity in rare cancers.
Long-read sequencing technologies: PacBio and Oxford nanopore
Long-read sequencing technologies, such as those developed by Pacific Biosciences (PacBio) and Oxford Nanopore, are transforming rare disease genomics. These platforms can sequence much longer stretches of DNA than traditional short-read methods, allowing for better detection of structural variants and repetitive regions that are often implicated in rare genetic disorders.
PacBio's Single Molecule, Real-Time (SMRT) sequencing and Oxford Nanopore's nanopore-based technology offer distinct advantages in rare disease research. They can resolve complex genomic regions, detect large insertions or deletions, and provide a more complete picture of the genome. This is particularly important for identifying de novo mutations and structural variations that may be missed by short-read sequencing.
Bioinformatics pipelines for rare variant detection
The wealth of data generated by genomic sequencing techniques requires sophisticated bioinformatics pipelines to identify rare variants that may be causative of disease. These computational tools are essential for filtering through the millions of genetic variations present in an individual's genome to pinpoint those most likely to be pathogenic.
Advanced algorithms and machine learning approaches are being developed to improve the accuracy of variant calling and interpretation. These tools consider factors such as allele frequency, evolutionary conservation, and predicted functional impact to prioritize variants for further investigation. Bioinformatics pipelines also integrate data from multiple sources, including population databases and functional studies, to provide a comprehensive assessment of variant pathogenicity.
High-throughput drug screening for rare disease therapeutics
High-throughput drug screening has become an invaluable tool in the search for treatments for rare diseases. This approach allows researchers to rapidly test thousands of compounds for their potential therapeutic effects, significantly accelerating the drug discovery process. By leveraging advanced technologies and automated systems, scientists can identify promising drug candidates more efficiently than ever before.
Induced pluripotent stem cell models in drug discovery
Induced pluripotent stem cells (iPSCs) have revolutionized the field of drug discovery for rare diseases. These cells, derived from adult tissues and reprogrammed to an embryonic-like state, can be differentiated into various cell types relevant to specific rare disorders. This allows researchers to create disease models that accurately reflect the genetic background of patients.
iPSC-based models offer several advantages in drug screening:
- They provide a renewable source of human cells for testing
- They can recapitulate disease-specific phenotypes
- They allow for personalized drug screening using patient-derived cells
- They reduce reliance on animal models, which may not always accurately represent human disease
By screening drug libraries on iPSC-derived cells, researchers can identify compounds that show promise in correcting disease-related cellular defects. This approach has already led to the discovery of potential treatments for rare conditions such as Familial Dysautonomia and Rett Syndrome.
Crispr-cas9 screening for target identification
CRISPR-Cas9 technology has emerged as a powerful tool for target identification in rare disease research. This gene-editing technique allows researchers to systematically knockout or modify genes in cells to understand their role in disease processes. When combined with high-throughput screening methods, CRISPR-Cas9 can rapidly identify genes that are essential for disease progression or potential therapeutic targets.
For example, CRISPR screens have been used to identify genes that confer resistance to certain therapies in rare cancers, leading to new combination treatment strategies. This approach is particularly valuable for rare diseases where traditional target identification methods may be limited by small patient populations.
Artificial intelligence in compound prediction and virtual screening
Artificial Intelligence (AI) and machine learning algorithms are increasingly being applied to drug discovery for rare diseases. These technologies can analyze vast amounts of data from previous drug screening efforts, scientific literature, and molecular databases to predict which compounds are most likely to be effective against a specific disease target.
AI-driven virtual screening offers several advantages:
- It can significantly reduce the number of compounds that need to be physically tested
- It can identify novel chemical structures that human researchers might overlook
- It can predict potential drug-target interactions and off-target effects
- It can optimize lead compounds for improved efficacy and reduced toxicity
For rare diseases, where the number of known disease-modifying compounds may be limited, AI can help extrapolate from existing data to suggest new therapeutic approaches. This is particularly valuable in repurposing existing drugs for rare disease indications, a strategy that can significantly reduce development time and costs.
Phenotypic screening approaches for rare disease drug development
Phenotypic screening has reemerged as a valuable approach in rare disease drug discovery. Unlike target-based screening, which focuses on a specific molecular target, phenotypic screening looks for compounds that produce a desired change in cellular or organismal phenotype. This is particularly useful for rare diseases where the underlying molecular mechanisms may not be fully understood.
Advanced imaging technologies and high-content screening platforms have made it possible to conduct phenotypic screens on a large scale. For example, zebrafish models of rare diseases can be used to screen thousands of compounds for their effects on complex developmental phenotypes. This approach has led to the discovery of potential treatments for rare conditions such as Duchenne Muscular Dystrophy and Dravet Syndrome.
Collaborative research initiatives and data sharing platforms
The complexity and rarity of many genetic disorders necessitate collaborative efforts on a global scale. Research initiatives and data sharing platforms have become crucial in advancing our understanding of rare diseases and accelerating the development of new therapies. These collaborative approaches allow researchers to pool resources, share expertise, and leverage large datasets that would be impossible to generate individually.
The international rare diseases research consortium (IRDiRC)
The International Rare Diseases Research Consortium (IRDiRC) is a prime example of a global collaborative effort in rare disease research. Established in 2011, IRDiRC brings together researchers, funders, companies, and patient organizations from around the world with the shared goal of accelerating progress in rare disease research and therapy development.
IRDiRC's ambitious goals include:
- Developing 1000 new therapies for rare diseases by 2027
- Diagnosing all patients with a rare disease within one year of coming to medical attention
- Developing methodologies to assess the impact of diagnoses and therapies on rare disease patients
By fostering international collaboration and setting shared research priorities, IRDiRC has helped to streamline research efforts and avoid duplication. The consortium also works to harmonize data collection and sharing practices, making it easier for researchers worldwide to access and analyze rare disease data.
Orphanet: reference portal for rare disease information
Orphanet is a comprehensive online resource that serves as a reference portal for information on rare diseases and orphan drugs. Established in 1997, Orphanet has become an invaluable tool for researchers, healthcare professionals, patients, and policymakers involved in the rare disease field.
Key features of Orphanet include:
- A comprehensive inventory of rare diseases, including detailed descriptions and gene associations
- A directory of expert centers, patient organizations, and clinical trials
- An encyclopedia of orphan drugs and ongoing research projects
- Tools for rare disease coding and classification
By centralizing and standardizing rare disease information, Orphanet facilitates research collaboration and helps to connect patients with relevant resources and ongoing studies. The platform's multi-lingual approach also ensures that this valuable information is accessible to a global audience.
Patient registries and biobanks for rare disease research
Patient registries and biobanks play a crucial role in rare disease research by collecting and storing valuable data and biological samples from affected individuals. These resources are essential for understanding the natural history of rare diseases, identifying potential biomarkers, and supporting clinical trials.
Patient registries collect standardized information about individuals with specific rare diseases, including:
- Demographic data
- Clinical symptoms and progression
- Genetic information
- Treatment outcomes
Biobanks, on the other hand, store biological samples such as blood, tissue, and DNA from patients with rare diseases. These samples are invaluable for genetic studies, biomarker discovery, and drug screening efforts.
The integration of patient registries and biobanks with other data sources, such as electronic health records and genomic databases, is creating powerful research platforms. For example, the European Reference Networks (ERNs) are working to establish disease-specific registries that will provide a comprehensive view of rare diseases across Europe.
DECIPHER: database for genomic variants and phenotypes
DECIPHER (Database of Chromosomal Imbalance and Phenotype in Humans using Ensembl Resources) is a web-based platform that facilitates the interpretation of genomic variants in rare diseases. This database allows researchers and clinicians to share and compare genomic and phenotypic data from patients with rare disorders.
Key features of DECIPHER include:
- A repository of anonymized patient data, including genetic variants and clinical features
- Tools for visualizing and analyzing genomic data
- Integration with other genomic resources and databases
- A platform for secure data sharing between clinicians and researchers
By enabling the comparison of rare genomic variants across a large number of patients, DECIPHER helps researchers identify new genotype-phenotype correlations and improve the interpretation of variants of uncertain significance. This collaborative approach is particularly valuable in the context of ultra-rare disorders, where individual institutions may only see a handful of cases.
Precision medicine approaches in rare disease treatment
Precision medicine holds great promise for the treatment of rare diseases, offering the potential for tailored therapies based on an individual's genetic makeup and specific disease characteristics. This approach is particularly well-suited to rare disorders, many of which have a clear genetic basis but may manifest differently in each patient.
Gene therapy strategies for monogenic rare disorders
Gene therapy has emerged as a groundbreaking approach for treating monogenic rare disorders, which are caused by mutations in a single gene. This technique involves delivering functional copies of the defective gene to affected cells or tissues, potentially correcting the underlying genetic cause of the disease.
Several gene therapy strategies are being explored for rare diseases:
- In vivo gene therapy, where the therapeutic gene is delivered directly to the patient's body
- Ex vivo gene therapy, where cells are modified outside the body and then reintroduced
- Gene editing approaches, such as CRISPR-Cas9, to correct mutations in situ
Recent successes in gene therapy for rare diseases include treatments for spinal muscular atrophy (SMA) and certain forms of inherited blindness. These breakthroughs demonstrate the potential of gene therapy to provide long-lasting or even curative treatments for previously untreatable conditions.
Antisense oligonucleotide therapies for rare neurological conditions
Antisense oligonucleotides (ASOs) are short, synthetic DNA-like molecules that can modulate gene expression. They have shown particular promise in treating rare neurological conditions, where traditional small molecule drugs often struggle to cross the blood-brain barrier.
Notable successes with ASO therapies include treatments for spinal muscular atrophy and Duchenne muscular dystrophy. The ability to rapidly design and synthesize ASOs tailored to specific genetic mutations makes this approach particularly attractive for ultra-rare disorders.
Small molecule chaperone therapy for lysosomal storage disorders
Small molecule chaperone therapy is an innovative approach for treating certain rare genetic disorders, particularly lysosomal storage diseases. These disorders are often caused by mutations that lead to misfolding of essential enzymes, resulting in their premature degradation or loss of function.
This approach has shown promise in disorders such as Fabry disease and Gaucher disease. Chaperone therapy offers several advantages over enzyme replacement therapy, including oral administration and the potential to cross the blood-brain barrier, making it suitable for treating neurological manifestations of lysosomal storage disorders.
Enzyme replacement therapy: successes and challenges
Enzyme replacement therapy (ERT) has been a groundbreaking approach for treating certain rare genetic disorders, particularly lysosomal storage diseases. This therapy involves administering recombinant enzymes to replace the deficient or dysfunctional enzymes in patients.
Key successes of enzyme replacement therapy include:
- Effective treatments for Gaucher disease, Fabry disease, and Pompe disease
- Improved quality of life and increased lifespan for many patients
- Reduction in organ damage and disease progression
- Relatively safe and well-tolerated treatment option
However, ERT also faces several challenges:
- High cost of treatment, often exceeding $100,000 per year per patient
- Need for frequent infusions, typically every 1-2 weeks
- Limited effectiveness in treating neurological symptoms due to poor blood-brain barrier penetration
- Potential for immune reactions to the recombinant enzymes
Despite these challenges, enzyme replacement therapy remains a crucial treatment option for many rare diseases. Ongoing research aims to improve enzyme delivery methods, enhance enzyme stability, and develop combination therapies to address the limitations of current ERT approaches.
Regulatory frameworks and incentives for orphan drug development
The development of treatments for rare diseases often faces unique challenges, including small patient populations and limited commercial potential. To address these issues and encourage investment in orphan drug development, regulatory agencies worldwide have implemented special frameworks and incentives.
Fda's orphan drug designation program
The U.S. Food and Drug Administration's Orphan Drug Designation Program provides several incentives to encourage the development of drugs for rare diseases. These incentives include:
- Seven years of market exclusivity upon approval
- Tax credits for clinical trial costs
- Waiver of Prescription Drug User Fee Act (PDUFA) filing fees
- Eligibility for research grants
To qualify for orphan drug designation, a drug must be intended to treat a condition affecting fewer than 200,000 people in the United States, or demonstrate that there is no reasonable expectation of recovering development costs through sales in the U.S. market. This program has been instrumental in stimulating research and development for rare disease treatments, with over 1,000 orphan drugs approved since the program's inception in 1983.
Ema's PRIME (PRIority MEdicines) scheme for rare diseases
The European Medicines Agency (EMA) launched the PRIME scheme in 2016 to support the development of medicines that target unmet medical needs. While not exclusive to rare diseases, PRIME is particularly relevant for orphan drug development. The scheme offers:
- Early and enhanced dialogue with regulators
- Scientific advice at key development milestones
- Potential for accelerated assessment of marketing authorization applications
- Guidance on clinical trial designs and data requirements
PRIME aims to optimize development plans and speed up evaluation processes, ensuring that promising therapies reach patients faster. For rare disease treatments, this support can be crucial in navigating the complex regulatory landscape and addressing the unique challenges of small patient populations.
Accelerated approval pathways for rare disease treatments
Recognizing the urgent need for treatments in many rare diseases, regulatory agencies have established accelerated approval pathways. These pathways allow for faster approval of drugs that address serious conditions with limited treatment options. Key features include:
- Approval based on surrogate endpoints or intermediate clinical endpoints
- Rolling review of application components as they become available
- Intensive guidance and support from regulatory agencies throughout the development process
Examples of accelerated approval pathways include the FDA's Breakthrough Therapy Designation and the EMA's Conditional Marketing Authorization. These pathways have been particularly beneficial for rare disease treatments, allowing patients earlier access to potentially life-saving therapies while additional confirmatory studies are conducted.
Tax credits and market exclusivity for orphan drug developers
To offset the financial risks associated with orphan drug development, many countries offer tax incentives and extended market exclusivity periods. These benefits include:
- Tax credits for clinical research expenses (up to 50% in the United States)
- Extended market exclusivity periods (7 years in the US, 10 years in the EU)
- Grants for clinical trials in rare diseases
- Fee waivers for regulatory submissions
These financial incentives play a crucial role in making rare disease research economically viable for pharmaceutical companies. By reducing the financial burden and extending the period during which companies can recoup their investments, these incentives have led to a significant increase in orphan drug development over the past few decades.